NCBI Bookshelf. A service of the National Library of Medicine, National Institutes of Health.
Liedtke WB, Heller S, editors. TRP Ion Channel Function in Sensory Transduction and Cellular Signaling Cascades. Boca Raton (FL): CRC Press/Taylor & Francis; 2007.
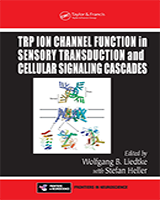
TRP Ion Channel Function in Sensory Transduction and Cellular Signaling Cascades.
Show detailsINTRODUCTION
We feel a wide range of temperatures spanning from coldness to heat. Within this range, temperatures over about 43°C and below about 15°C evoke not only a thermal sensation, but also a feeling of pain (LaMotte and Campbell, 1978; Tillman et al., 1995). Neurophysiological studies have demonstrated that the heat threshold of so-called C-fiber mechanoheat nociceptors (CMHs) depends on the absolute temperature, rather than the rate of temperature increase, and that the transduction of heat stimuli occurs at different skin depths for different CMHs (Tillman et al., 1995). Extreme cold also activates a subset of nociceptive neurons. However, the physiology of cold-evoked pain is not as well understood as that of heat-evoked pain. It has been hypothesized that cutaneous nociceptor endings detect temperature and other physical stimuli by means of ion channels responsive to these stimuli. The first support for this hypothesis came from the identification of heat-gated ion channels present in a subset of primary afferent neurons (Cesare and McNaughton, 1996; Reichling and Levine, 1997). Insight into the molecular nature of these channels came shortly thereafter, with the cloning of the capsaicin receptor, TRPV1 (also known as VR1, the first member of the TRPV subfamily) and the recognition that this ion channel protein, like mammalian nociceptors, could be activated by elevated temperatures with a discrete threshold near 43°C (Caterina et al., 1997; Caterina and Julius, 2001). Three other TRPV channels—TRPV2 (also known as VRL-1), TRPV3, and TRPV4 (also known as VROAC or OTRPC4)—have been cloned and characterized as heat or warm thermosensors (Jordt et al., 2003; Patapoutian et al., 2003; Tominaga and Caterina, 2004). In addition, two TRPM channels (TRPM4 and TRPM5) have been recently reported to be thermosensitive (Talavera et al., 2005). The threshold temperatures for activation of these channels range from relatively warm (TRPV3, TRPV4, TRPM4, and TRPM5) to extremely hot (TRPV2). In contrast to these warmth- or heat-activated TRP channels, two other TRP channels—TRPM8 (also known as CMR1) and TRPA1 (also known as ANKTM1)—are activated by cold stimuli (Jordt et al., 2003; Patapoutian et al., 2003; Tominaga and Caterina, 2004). This chapter focuses on eight mammalian thermosensitive TRP channels (Figure 20.1).
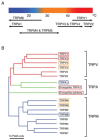
FIGURE 20.1
(A) Temperature ranges activating thermosensitive TRP channels. (B) Phylogenetic relationship among the mammalian TRPV, TRPM, and TRPA channels with two Drosophila TRPA channels. Red, orange, and blue squares indicate channels activated by high heat, (more...)
HEAT RECEPTORS
TRPV1 (VR1)
When a receptor for capsaicin, a main pungent ingredient of hot chili peppers, was isolated using a Ca2+-imaging–based expression cloning method in 1997, it was designated vanilloid receptor subtype 1 (VR1), then renamed as TRPV1 (Caterina et al., 1997; Caterina and Julius, 2001). Patch-clamp recordings from human embryonic kidney-derived HEK293 cells transfected with TRPV1 cDNA (Caterina et al., 1997; Tominaga et al., 1998) revealed that capsaicin-activated TRPV1 currents exhibit nonselective cation permeability with an outwardly-rectifying current-voltage (I-V) relationship. TRPV1 was also found to show high Ca2+ permeability (PCa/PNa= 9.6), which may be one of the reasons why the Ca2+-imaging strategy successfully cloned this molecule. Application of capsaicin to membrane patches excised from HEK293 cells expressing TRPV1 was shown to evoke clear single-channel openings (conductance of ~77 pS for Na+), strongly suggesting that no cytosolic second messengers are necessary for TRPV1 activation.
TRPV1 transcript and protein were found to be most highly expressed in sensory neurons, especially in small diameter neurons within dorsal root and trigeminal sensory ganglia (DRG and TG, respectively), probably cell bodies of unmyelinated C-fibers (Caterina et al., 1997; Tominaga et al., 1998), consistent with the notion that polymodal C-fibers are involved in nociception. The burning quality of capsaicin-induced pain suggests that capsaicin and heat may evoke painful responses through a common molecular pathway. Consistent with this hypothesis, TRPV1 was found to be activated by heat at >43°C, a temperature threshold similar to that at which heat evokes pain in vivo (Caterina et al., 1997; Tominaga et al., 1998). Single-channel openings recorded in excised-membrane patches expressing TRPV1 suggested that heat gates TRPV1 directly and that TRPV1 is itself a heat sensor. These findings also suggested that TRPV1 might constitute the heat-activated ion channels that have been characterized electrophysiologically in cultured sensory neurons and found to be sensitized through the protein kinase C-ɛ dependent pathway (Cesare et al., 1999). A strong correlation between nociceptor capsaicin responsiveness and heat responsiveness (Kirschstein et al., 1997; Nagy and Rang, 1999) provided further support for this idea.
Additional studies demonstrated that TRPV1 could alternatively be activated at room temperature when the proton concentration was increased (< pH 6.0), and that such proton-evoked TRPV1 activation was observed in excised-membrane patches, indicating that protons gate TRPV1 directly (Tominaga et al., 1998). Thus, TRPV1 can be activated by at least three different pain-producing stimuli: capsaicin, heat (>43°C), or protons. These stimuli are likely to work in concert to regulate the activity of TRPV1 in vivo, especially under pathological conditions where tissue acidosis and elevated temperature may come into play.
The studies outlined above provided in vitro evidence that TRPV1 serves as a transducer of noxious thermal and chemical stimuli in nociceptors. To determine whether TRPV1 really contributes to the detection of these noxious stimuli in vivo, mice lacking this protein were generated and analyzed for nociceptive function. Sensory neurons from mice lacking TRPV1 were deficient in their responses to each of the reported noxious stimuli: capsaicin, protons, and heat (Caterina et al., 2000; Davis et al., 2000). Consistent with this observation, behavioral responses to capsaicin were absent and responses to acute thermal stimuli were diminished in these mice. The most prominent feature of the knockout mouse thermosensory phenotype was a virtual absence of thermal hypersensitivity in the setting of inflammation. These findings indicate that TRPV1 is essential for selective modalities of pain sensation and for tissue injury–induced thermal hyperalgesia. In addition, fever production in response to the bacterial pyrogen, lipopolysaccharide, was significantly attenuated in TRPV1-deficient mice, although its molecular mechanism is not known (Iida et al., 2005). The extent to which TRPV1 underlies the responses to noxious thermal stimuli and the contribution of other heat-sensitive channels remains to be clarified. There was a drastic reduction of heat sensitivity in DRG neurons cultured from TRPV1-deficient mice, but a small yet significant percentage of DRG neurons showed large heat-evoked current responses to heat stimuli over 55°C. Furthermore, the TRPV1-deficient mice showed impaired responses to noxious thermal stimuli only over 50°C, and a small but significant amount of heat-evoked c-Fos induction persisted in spinal cord laminae I and II of TRPV1-deficient mice (Caterina et al., 2000). These data supported the idea that other heat-sensitive channels contribute to the transmission and perception of high-intensity noxious thermal stimuli.
Inflammatory pain is initiated by tissue damage/inflammation and is characterized by hypersensitivity both at the site of damage and in adjacent tissue. Stimuli that normally would not produce pain do so (allodynia), while previously noxious stimuli evoke even greater pain responses (hyperalgesia). One mechanism underlying these phenomena is the modulation (sensitization) of ion channels such as TRPV1 (Mizumura and Kumazawa, 1996; Wood and Perl, 1999; Woolf and Salter, 2000). Sensitization is triggered by extracellular inflammatory mediators that are released in vivo from surrounding damaged or inflamed tissues and from nociceptive neurons themselves (i.e., neurogenic inflammation). Mediators known to cause sensitization include prostaglandins, adenosine, serotonin, bradykinin, and ATP (Julius and Basbaum, 2001). Among the inflammatory mediators, extracellular ATP, bradykinin, prostagladins (prostaglandin E2 and prostaglandin I2), and trypsin or tryptase have been reported to potentiate TRPV1 responses through metabotropic P2Y2, B1 or B2, EP1 or IP, and proteinase-activated receptor-2 (PAR-2) receptors, respectively, in a PKC-dependent manner in both a heterologous expression system and native DRG neurons (Tominaga et al., 2001; Sugiura et al., 2002; Moriyama et al., 2003; Amadesi et al., 2004; Dai et al., 2004; Moriyama et al., 2005). In addition to potentiating capsaicin- or proton-evoked currents, ATP, bradykinin, prostaglandin E2, prostaglandin I2, trypsin, or tryptase also lower the temperature threshold for heat activation of TRPV1 to as low as 30°C, such that normally nonpainful thermal stimuli (i.e., normal body temperature) can activate TRPV1. Under these circumstances, those inflammatory mediators thus resemble direct activators of TRPV1. These inflammatory mediator-induced TRPV1-mediated hypersensitivities were confirmed at the whole animal level using TRPV1-deficient mice or mice lacking receptors for inflammatory mediators (Moriyama et al., 2003; Dai et al., 2004; Moriyama et al., 2005). PKC-dependent phosphorylation of TRPV1 has been found to be involved in the sensitization of TRPV1 by the inflammatory mediators. Indeed, two serine residues in the cytoplasmic domain of TRPV1 were identified as substrates for PKC-dependent phosphorylation (Numazaki et al., 2002; Bhave et al., 2003). Although other pathways including a PKA-dependent one were found to be involved in sensitizing TRPV1, the PKC-dependent pathway seems to be predominantly involved in reducing the temperature threshold for TRPV1 activation. Tissue acidification is induced in pathological conditions such as ischemia or inflammation (Bevan and Geppetti, 1994), and such acidification exacerbates or causes pain. In addition to the direct activation of TRPV1, acidification also shifts the temperature-response curve of TRPV1 to the left so that the channel can be activated at lower temperatures (lower than body temperature), and responses to heat are bigger at a given suprathreshold temperature (Tominaga et al., 1998). This phenomenon might also contribute to inflammatory pain.
How can heat open this channel? A Q10 value of ~26 for TRPV1 far surpasses the temperature dependence of the gating processes characterized by other ion channels (Q10 ~3). The distal half of the TRPV1 carboxyl terminus was reported to be partially involved in thermal sensitivity (Vlachova et al., 2003). TRPV1 is known to have a voltage-dependent gating property (Gunthorpe et al., 2000). Nilius and colleagues have reported that temperature sensing in TRPV1 and TRPM8 (described later) is tightly linked to voltage-dependent gating (Voets et al., 2004). TRPV1 is activated upon depolarization, and changes in temperature result in graded shifts in its voltage-dependent activation curve. Through mathematical modeling, Nilius et al. made a single thermodynamic model that shows how temperature changes the thermal responsiveness of both channels. This suggests that amino acids responsible for voltage dependence are also involved in thermosensing, although the fourth TM domain of TRPV1 lacks the multiple positively charged residues typical of voltage-gated channels.
TRPV2 (VRL-1)
A protein with 49 percent of the same qualities as TRPV1 was isolated and designated vanilloid-receptor-like protein 1 (VRL-1) and later renamed TRPV2 (Caterina et al., 1999). TRPV2 is not activated by vanilloids, protons, or moderate thermal stimuli but can be activated by high temperatures with a threshold of ~52°C. TRPV2 currents showed similar properties to those of TRPV1, such as an outwardly rectifying I-V relationship at positive potentials, inhibition by ruthenium red, and relatively high Ca2+ permeability (PCa/PNa= 2.9). However, whether TRPV2 is gated directly by high heat at a single-channel level is not known, probably because thermal noise inevitably contaminates recordings at such high temperatures.
Intense TRPV2 immunoreactivity was observed in medium- to large-diameter cells in rat DRG neurons (Caterina et al., 1999; Ma, 2001; Ahluwalia et al., 2002; Lewinter et al., 2004). Many of the TRPV2 immunoreactive cells in rat DRG were co-stained with the anti-neurofilament antibody N52, a marker for myelinated neurons. Dense TRPV2 immunoreactivity in the spinal cord was found in lamina I, inner lamina II, and laminae III/IV. This is consistent with the expression of TRPV2 in myelinated nociceptors that target laminae I and inner lamina II and in non-nociceptive Aβ fibers that target laminae III/IV. Aδ mechano- and heat-sensitive (AMH) neurons in monkeys are medium- to large-diameter, lightly myelinated neurons that fall into two groups: type I AMHs have a heat threshold of ~53°C, and type II AMHs are activated at 43°C (Treede et al., 1995). The TRPV2 localization data and the residual high temperature–evoked responses observed in the TRPV1-deficient mice suggest that TRPV2 expression might account for the high thermal threshold ascribed to type I AMH nociceptors. Temperatures activating TRPV2 are more harmful to our body than those activating TRPV1. Therefore, TRPV2 expression in the myelinated sensory fibers seems reasonable because Aδ fibers can transmit nociceptive information much faster than C fibers, which exclusively express TRPV1. In addition to the acute thermal nociception, TRPV2 was suggested to be involved in peripheral sensitization during inflammation based on the result that injection of complete Freund’s adjuvant induced upregulation of TRPV2 expression in rat DRG neurons (Shimosato et al., 2005). The function of TRPV2 in Aβ fibers is not known.
TRPV2 transcript and protein were found not only in sensory neurons but also in motoneurons and in many nonneuronal tissues that are unlikely to be exposed to temperatures above 50°C (Caterina et al., 1999; Lewinter et al., 2004). These results indicate that TRPV2 undoubtedly contributes to numerous functions in addition to nociceptive processing. Indeed, growth factor-regulated channel, GRC (the likely mouse TRPV2 orthologue), was reported to translocate from cytosol to the plasma membrane upon stimulation with insulinlike growth factor (Kanzaki et al., 1999). And, mouse GRC has been found to be activated by stretching in cardiac myocytes (Iwata et al., 2003), suggesting that TRPV2 may be activated by mechanical stimuli.
WARM RECEPTORS
TRPV3 and TRPV4
TRPV3 and TRPV4 (also known as VROAC or OTRPC4 from vanilloid receptor–related osmotically activated channel or OSM-9-like TRP channel 4, respectively) have been found to be activated by warm temperatures (~34–38°C for TRPV3 and ~27–35°C for TRPV4) in heterologous expression systems, and to be expressed in multiple tissues including, among others, sensory and hypothalamic neurons and keratinocytes (Guler et al., 2002; Peier, Reeve, et al., 2002; Smith et al., 2002; Watanabe et al., 2002; Xu et al., 2002). TRPV4 can alternatively be activated by nonthermal stimuli, including hypoosmolarity (Liedtke et al., 2000; Strotmann et al., 2000; Wissenbach et al., 2000), certain synthetic phorbol esters, and 5’,6’-epoxye-icosatrienoic acid (Watanabe et al., 2002; Watanabe, Vriens, et al., 2003). However, TRPV4 activation by heat was observed in a whole-cell configuration but not in excised patches, suggesting the involvement of some cytosolic molecules in its activation, a different mechanism from that of TRPV1. Several approaches, including the knockdown of TRPV4 with gene disruption or antisense oligonucleotides, have led to reports that this protein is involved in mechanical stimulus– and hypotonicity-induced nociception in rodents at baseline or following hypersensitivity induced by prostaglandin injection or taxol neurotoxicity (Alessandri-Haber et al., 2003; Suzuki et al., 2003; Alessandri-Haber et al., 2004).
In terms of the thermosensing ability of TRPV4 in vivo, peripheral nerve recordings suggested that there might be a decrease in warmth-evoked electrical activity in TRPV4-deficient mice (Todaka et al., 2004). Moreover, both TRPV3 and TRPV4 have been reported to function in thermosensation by keratinocytes, based on studies of wild-type and TRPV4-deficient keratinocytes (Chung et al., 2003; Chung et al., 2004). In contrast, no changes in escape latency from heat stimuli were observed in either the hot plate (Suzuki et al., 2003; Todaka et al., 2004) or radiant paw heating (Liedtke and Friedman, 2003) assays. After subcutaneous injection of capsaicin or carrageenan, however, TRPV4-deficient mice showed longer escape latencies from a hot surface, relative to wild-type controls (Todaka et al., 2004). Thus, the precise contributions of TRPV4 to thermosensation and thermoregulation in vivo seem unclear. However, experiments using a thermal gradient apparatus revealed that TRPV4-deficient mice selected warmer floor temperatures than wild-type mice (Lee et al., 2005). In addition, whereas wild-type mice failed to discriminate between floor temperatures of 30 and 34°C, TRPV4-deficient mice exhibited a strong preference for 34°C and prolonged withdrawal latencies during acute tail heating. These results indicate that TRPV4 is required for normal thermal responsiveness. Further, the fact that TRPV4 is expressed in the hypothalamus, a center for body temperature regulation, suggests that TRPV4 is involved in thermoregulation in the brain by detecting temperature directly as well.
TRPV3-deficient mice lost preference for 35°C compared with room temperature, which was evident in wild-type mice, suggesting an important role for TRPV3 in innocuous thermosensation (Moqrich et al., 2005). In addition, TRPV3-deficient mice exhibited delayed responses in tail flick assay (over 50°C) and hot plate tests (over 55°C), indicating that TRPV3 is involved in thermal nociception, consistent with the sensitization of TRPV3 upon repeated noxious heat stimuli (Peier, Reeve, et al., 2002). The latter thermal nociceptive phenotype in TRPV3-deficient mice is similar to those reported for TRPV1-deficient mice, suggesting that these two TRPV channels have overlapping functions in vivo. The former innocuous thermal phenotype apparently seems to contradict one observed for TRPV4-deficient mice. Functional discrimination of the two thermosensitive TRP channels with similar temperature activation thresholds in keratinocytes and the possible signal transduction from keratinocytes to sensory neurons would be important research directions in the future. Inflammatory mediator-induced thermal hyperalgesia was reported to be indistinguishable between wild-type and TRPV3-deficient mice (Moqrich et al., 2005).
TRPM4 and TRPM5
TRPM4 is expressed in many tissues and cell types, and it functions as a Ca2+-activated nonselective cation channel (Launay et al., 2002). Full-length TRPM4 (designated TRPM4b to distinguish it from the shorter TRPM4a variant) carries monovalent cations. Single-channel I-V relationship of TRPM4 is linear with a slope conductance of ~25 pS, although voltage-dependent modulation causes an outwardly rectifying steady-state I-V relationship (Nilius et al., 2003). The gene encoding TRPM5 was identified during functional analysis of a chromosomal region that is associated with several tumors (Prawitt et al., 2000). TRPM5 is activated directly by elevated Ca2+ (Hofmann et al., 2003; Prawitt et al., 2003), and it was reported to be activated in the downstream of activation of G-protein-coupled taste receptors (Perez et al., 2002). Like TRPM4, TRPM5 is a monovalent-specific ion channel with ~25 pS conductance and has the steady-state I-V relationship characterized by strong outward rectification.
TRPM4 and TRPM5 have been found to be temperature-sensitive, heat-activated ion channels (Talavera et al., 2005). TRPM4- or TRPM5-mediated inward currents increased steeply at temperatures between 15 and 35°C. Heat activation was due to a temperature-dependent shift of the activation curve, in analogy to TRPV1. Also, increasing temperature between 15 and 35°C markedly enhanced the gustatory nerve response to sweet compounds in wild-type mice but not in mice lacking TRPM5, suggesting that temperature sensitivity of TRPM5 may underlie known effects of temperature on perceived taste in humans (Bartoshuk et al., 1982; Green and Frankmann, 1988).
COLD RECEPTORS
TRPM8 (CMR1)
A distinct class of cold-sensitive fibers has been described as polymodal nociceptors, responding to noxious cold, heat, and pinching (Campero et al., 1996; Simone and Kajander, 1997; Cain et al., 2001), although an early study suggested a distinction between cold sensation and painful sensation (Klement and Arndt, 1992). The cooling sensation of menthol, a chemical agent found in mint, is well established (Hensel and Zoterman, 1951), and both cooling and menthol have been suggested to be transduced through a nonselective cation channel in DRG neurons (Reid and Flonta, 2001; Reid et al., 2002). Two groups independently cloned and characterized a cold receptor, TRPM8 (also known as CMR1, for cold- and menthol-sensitive receptor 1), which can also be activated by menthol (McKemy et al., 2002; Peier, Moqrich, et al., 2002). In heterologous expression systems, TRPM8 could be activated by menthol or by cooling, with an activation temperature of ~25–28°C. TRPM8 could alternatively be activated by other cooling compounds, such as menthone, eucalyptol, and icilin. There also appears to be interaction between effective stimuli for TRPM8, in that subthreshold concentrations of menthol increased the temperature threshold for TRPM8 activation from 25°C to 30°C. This is reminiscent of TRPV1, whose activation temperature is reduced under mildly acidic conditions that do not open TRPV1 alone (Tominaga et al., 1998). Whole-cell recording in HEK293 cells expressing TRPM8 revealed that TRPM8 is a nonselective cation channel with relatively high Ca2+ permeability (PCa/PNa= 3.3) and that TRPM8 shows an outwardly rectifying I-V relationship like TRPV1. Single-channel recordings showed a conductance of 83 pS at positive potentials. Cold activation of TRPM8 was reported to be due to a temperature-dependent shift of the activation curve, in analogy to TRPV1, TRPM4, and TRPM5 (Voets et al., 2004).
TRPM8 is expressed in a subset of DRG and TG neurons that can be classified as small-diameter C fibers (McKemy et al., 2002; Peier, Moqrich, et al., 2002; Nealen et al., 2003). Interestingly, however, TRPM8 is not coexpressed with TRPV1, which marks a class of nociceptors. Whether TRPM8 is involved in cold nociception remains to be clarified, although inflammatory mediators were found to downregulate TRPM8 via PKC-mediated phosphorylation (Premkumar et al., 2005), suggesting that the TRPM8 downregulation might aggravate thermal hyperalgesia in the context of inflammation. Regarding channel regulation, reciprocal modulation of TRPM8 and TRPV1 by PIP2 has been described (Chuang et al., 2001; Liu and Qin, 2005; Rohacs et al., 2005). TRPM8 is activated, whereas TRPV1 is tonically inhibited by PIP2. Hydrolysis of PIP2 by activating phospholipase C downregulates TRPM8 function and upregulates TRPV1 function. Bradykinin-induced downregulation of TRPM8 and upregulation of TRPV1 could almost completely be reversed by a PKC inhibitor (Premkumar et al., 2005). Thus, this mechanism of TRPM8 downregulation could worsen inflammatory hyperalgesia because more cooling would be necessary to activate TRPM8.
TRPA1 (ANKTM1)
TRPA1 (also known as ANKTM1, a channel containing both N-terminal ankyrin repeat domains and six transmembrane domains) was reported as a distantly related TRP channel that is activated by cold with a lower activation threshold as compared to TRPM8 (Story et al., 2003). In heterologous expression systems, TRPA1 was activated by cold stimuli with an activation temperature of about 17°C, which is close to the reported noxious cold threshold. This led to the suggestion that TRPA1 is involved in cold nociception. Indeed, TRPA1 has been involved in cold hyperalgesia caused by inflammation or nerve injury via activation of MAP kinase (Obata et al., 2005). Whole-cell recording in fibroblastic CHO cells expressing TRPA1 revealed cationic permeability with similar preferences for monovalent and divalent cations (PCa/PNa= 0.8) and an outwardly rectifying I-V relationship. Whether TRPA1 is gated directly by cold remains to be elucidated because no single-channel data have been reported. A recent study from another group failed to reproduce cold responsiveness in TRPA1 (Jordt et al., 2004). The reason for this apparent discrepancy is unclear. However, both groups have demonstrated that TRPA1 can be activated by pungent isothiocyanate compounds such as those found in wasabi, horse-radish, and mustard oil (Bandell et al., 2004; Jordt et al., 2004) or by allicin, a pungent ingredient of garlic (Bautista et al., 2005; Macpherson et al., 2005). Thus, several of the thermosensitive TRP channels likely to be involved in nociception can be activated by stimuli other than temperature (Table 20.1).
TABLE 20.1
The Eight Thermosensitive TRP Channels
Unlike TRPM8, TRPA1 is specifically expressed in a subset of sensory neurons that express the nociceptive markers CGRP and substance P (Story et al., 2003). Furthermore, TRPA1 is frequently coexpressed with TRPV1, raising the possibility that TRPA1 and TRPV1 mediate the function of a class of polymodal nociceptors. Such coexpression might also explain the paradoxical hot sensation experienced when one is exposed to a very cold stimulus.
THERMAL NOCICEPTION THROUGH TRP CHANNELS IN INVERTEBRATES
Invertebrates, like vertebrates, need to detect environmental temperature for their survival and to escape potentially dangerous stimuli. Interestingly, recent experiments indicate that TRP channels play a critical role in thermosensation in invertebrate, as well as vertebrate, species (Goodman, 2003; Zars, 2003). A TRPA1 homologue identified in Drosophila, named Painless, is expressed in thermosensory neurons and was found to be required for withdrawal responses to noxious thermal and mechanical stimuli. Although it is not clear whether Painless senses temperature directly (Tracey et al., 2003), it may be noteworthy that the threshold for evoking Painless-dependent behavior in Drosophila larvae is approximately 39–42°C, similar to the threshold for thermal pain in mammals. Moreover, residual withdrawal responses to a 52°C stimulus are preserved in painless mutants, suggesting that Drosophila, like mammals, may possess multiple mechanisms for thermonociception. Another recent study showed that the apparent Drosophila ortholog of TRPA1 (not Painless) is activated by warm temperatures (about 24–29°C) when expressed heterologously (Viswanath et al., 2003). Given that these species prefer an ambient temperature of ~24°C, this warmth-sensitive TRPA1 orthologue might also participate in some form of aversive heat sensation. It is particularly intriguing that the mammalian and Drosophila TRPA1 orthologues, while similar in primary sequence, are activated by two apparently opposite stimuli: cold and warmth. The mechanistic basis of this difference remains to be determined.
CONCLUSION
Significant advances in thermosensation and thermoregulation research have been made in the last several years with the cloning and characterization of thermosensitive TRP channels. With these clones, we can understand thermosensation and thermoregulation from a molecular standpoint. How temperature gates TRP channels is a very interesting question, but is not answered yet. Chemical agonists have been identified for many of the thermosensitive TRP channels (TRPV1, TRPV2, TRPV3, TRPV4, TRPM8, and TRPA1) (Table 20.1). Understanding the mechanisms of direct or indirect TRP channel opening by chemical activators might clarify the gating mechanism by thermal stimulation. It is also not known how multiple thermosensitive TRP channels are used within the same or different sensory neurons to provide us with the ability to evaluate temperature precisely over a broad range. Central integration of the thermal information acquired by peripheral nerve endings is almost certainly a critical component of this process. In addition, function of thermosensitive TRP channels in tissues other than neurons will have to be extensively investigated. Furthermore, regulatory mechanisms similar to those emerging for TRPV1 are likely to exist for most of the thermosensitive TRP channels. Therefore, an understanding of the molecules surrounding thermosensitive TRP channels will be indispensable to a complete understanding of thermosensation and thermoregulation.
REFERENCES
- Ahluwalia J, Rang H, Nagy I. The putative role of vanilloid receptor-like protein-1 in mediating high-threshold noxious heat-sensitivity in rat cultured primary sensory neurons. Eur J Neurosci. 2002;16:1483–1489. [PubMed: 12405961]
- Alessandri-Haber N, Dina OA, Yeh JJ, Parada CA, Reichling DB, Levine JD. Transient receptor potential vanilloid 4 is essential in chemotherapy-induced neuropathic pain in the rat. J Neurosci. 2004;24:4444–4452. [PMC free article: PMC6729449] [PubMed: 15128858]
- Alessandri-Haber N, Yeh JJ, Boyd AE, Parada CA, Chen X, Reichling DB, Levine JD. Hypotonicity induces TRPV4-mediated nociception in rat. Neuron. 2003;39:497–511. [PubMed: 12895423]
- Amadesi S, Nie J, Vergnolle N, Cottrell GS, Grady EF, Trevisani M, Manni C, Geppetti P, McRoberts JA, Ennes H, Davis JB, Mayer EA, Bunnett NW. Protease-activated receptor 2 sensitizes the capsaicin receptor transient receptor potential vanilloid receptor 1 to induce hyperalgesia. J Neurosci. 2004;24:4300–4312. [PMC free article: PMC6729438] [PubMed: 15128844]
- Bandell M, Story GM, Hwang SW, Viswanath V, Eid SR, Petrus MJ, Earley TJ, Patapoutian A. Noxious cold ion channel TRPA1 is activated by pungent compounds and bradykinin. Neuron. 2004;41:849–857. [PubMed: 15046718]
- Bartoshuk LM, Rennert K, Rodin J, Stevens JC. Effects of temperature on the perceived sweetness of sucrose. Physiol Behav. 1982;28:905–910. [PubMed: 7100291]
- Bautista DM, Movahed P, Hinman A, Axelsson HE, Sterner O, Hogestatt ED, Julius D, Jordt SE, Zygmunt PM. Pungent products from garlic activate the sensory ion channel TRPA1. Proc Natl Acad Sci USA. 2005;102:12248–12252. [PMC free article: PMC1189336] [PubMed: 16103371]
- Bevan S, Geppetti P. Protons: small stimulants of capsaicin-sensitive sensory nerves. Trends Neurosci. 1994;17:509–512. [PubMed: 7532332]
- Bhave G, Hu HJ, Glauner KS, Zhu W, Wang H, Brasier DJ, Oxford GS, Gereau RWt. Protein kinase C phosphorylation sensitizes but does not activate the capsaicin receptor transient receptor potential vanilloid 1 (TRPV1). Proc Natl Acad Sci USA. 2003;100:12480–12485. [PMC free article: PMC218783] [PubMed: 14523239]
- Cain DM, Khasabov SG, Simone DA. Response properties of mechanoreceptors and nociceptors in mouse glabrous skin: an in vivo study. J Neurophysiol. 2001;85:1561–1574. [PubMed: 11287480]
- Campero M, Serra J, Ochoa JL. C-polymodal nociceptors activated by noxious low temperature in human skin. J Physiol. 1996;497(Pt 2):565–572. [PMC free article: PMC1161005] [PubMed: 8961196]
- Caterina MJ, Julius D. The vanilloid receptor: a molecular gateway to the pain pathway. Annu Rev Neurosci. 2001;24:487–517. [PubMed: 11283319]
- Caterina MJ, Leffler A, Malmberg AB, Martin WJ, Trafton J, Petersen-Zeitz KR, Koltzenburg M, Basbaum AI, Julius D. Impaired nociception and pain sensation in mice lacking the capsaicin receptor. Science. 2000;288:306–313. [PubMed: 10764638]
- Caterina MJ, Rosen TA, Tominaga M, Brake AJ, Julius D. A capsaicin-receptor homologue with a high threshold for noxious heat. Nature. 1999;398:436–441. [PubMed: 10201375]
- Caterina MJ, Schumacher MA, Tominaga M, Rosen TA, Levine JD, Julius D. The capsaicin receptor: a heat-activated ion channel in the pain pathway. Nature. 1997;389:816–824. [PubMed: 9349813]
- Cesare P, Dekker LV, Sardini A, Parker PJ, McNaughton PA. Specific involvement of PKC-epsilon in sensitization of the neuronal response to painful heat. Neuron. 1999;23:617–624. [PubMed: 10433272]
- Cesare P, McNaughton P. A novel heat-activated current in nociceptive neurons and its sensitization by bradykinin. Proc Natl Acad Sci USA. 1996;93:15435–15439. [PMC free article: PMC26422] [PubMed: 8986829]
- Chuang HH, Prescott ED, Kong H, Shields S, Jordt SE, Basbaum AI, Chao MV, Julius D. Bradykinin and nerve growth factor release the capsaicin receptor from PtdIns(4,5)P2-mediated inhibition. Nature. 2001;411:957–962. [PubMed: 11418861]
- Chung MK, Lee H, Caterina MJ. Warm temperatures activate TRPV4 in mouse 308 keratinocytes. J Biol Chem. 2003;278:32037–32046. [PubMed: 12783886]
- Chung MK, Lee H, Mizuno A, Suzuki M, Caterina MJ. TRPV3 and TRPV4 mediate warmth-evoked currents in primary mouse keratinocytes. J Biol Chem. 2004;279:21569–21575. [PubMed: 15004014]
- Dai Y, Moriyama T, Higashi T, Togashi K, Kobayashi K, Yamanaka H, Tominaga M, Noguchi K. Proteinase-activated receptor 2-mediated potentiation of transient receptor potential vanilloid subfamily 1 activity reveals a mechanism for proteinase-induced inflammatory pain. J Neurosci. 2004;24:4293–4299. [PMC free article: PMC6729433] [PubMed: 15128843]
- Davis JB, Gray J, Gunthorpe MJ, Hatcher JP, Davey PT, Overend P, Harries MH, Latcham J, Clapham C, Atkinson K, Hughes SA, Rance K, Grau E, Harper AJ, Pugh PL, Rogers DC, Bingham S, Randall A, Sheardown SA. Vanilloid receptor-1 is essential for inflammatory thermal hyperalgesia. Nature. 2000;405:183–187. [PubMed: 10821274]
- Goodman MB. Sensation is painless. Trends Neurosci. 2003;26:643–645. [PubMed: 14624845]
- Green BG, Frankmann SP. The effect of cooling on the perception of carbohydrate and intensive sweeteners. Physiol Behav. 1988;43:515–519. [PubMed: 3194473]
- Guler AD, Lee H, Iida T, Shimizu I, Tominaga M, Caterina M. Heat-evoked activation of the ion channel, TRPV4. J Neurosci. 2002;22:6408–6414. [PMC free article: PMC6758176] [PubMed: 12151520]
- Gunthorpe MJ, Harries MH, Prinjha RK, Davis JB, Randall A. Voltage- and time-dependent properties of the recombinant rat vanilloid receptor (rVR1). J Physiol. 2000;525(Pt 3):747–759. [PMC free article: PMC2269971] [PubMed: 10856126]
- Hensel H, Zoterman Y. The effect of menthol on the thermoreceptors. Acta Physiol Scand. 1951;24:27–34. [PubMed: 14877581]
- Hofmann T, Chubanov V, Gudermann T, Montell C. TRPM5 is a voltage-modulated and Ca(2+)-activated monovalent selective cation channel. Curr Biol. 2003;13:1153–1158. [PubMed: 12842017]
- Iida T, Shimizu I, Nealen ML, Campbell A, Caterina M. Attenuated fever response in mice lacking TRPV1. Neurosci Lett. 2005;378:28–33. [PubMed: 15763167]
- Iwata Y, Katanosaka Y, Arai Y, Komamura K, Miyatake K, Shigekawa M. A novel mechanism of myocyte degeneration involving the Ca2+-permeable growth factor–regulated channel. J Cell Biol. 2003;161:957–967. [PMC free article: PMC2172975] [PubMed: 12796481]
- Jordt SE, Bautista DM, Chuang HH, McKemy DD, Zygmunt PM, Hogestatt ED, Meng ID, Julius D. Mustard oils and cannabinoids excite sensory nerve fibres through the TRP channel ANKTM1. Nature. 2004;427:260–265. [PubMed: 14712238]
- Jordt SE, McKemy DD, Julius D. Lessons from peppers and peppermint: the molecular logic of thermosensation. Curr Opin Neurobiol. 2003;13:487–492. [PubMed: 12965298]
- Julius D, Basbaum AI. Molecular mechanisms of nociception. Nature. 2001;413:203–210. [PubMed: 11557989]
- Kanzaki M, Zhang YQ, Mashima H, Li L, Shibata H, Kojima I. Translocation of a calcium-permeable cation channel induced by insulin-like growth factor-I. Nat Cell Biol. 1999;1:165–170. [PubMed: 10559903]
- Kirschstein T, Busselberg D, Treede RD. Coexpression of heat-evoked and capsaicin-evoked inward currents in acutely dissociated rat dorsal root ganglion neurons. Neurosci Lett. 1997;231:33–36. [PubMed: 9280161]
- Klement W, Arndt JO. The role of nociceptors of cutaneous veins in the mediation of cold pain in man. J Physiol. 1992;449:73–83. [PMC free article: PMC1176068] [PubMed: 1522527]
- LaMotte RH, Campbell JN. Comparison of responses of warm and nociceptive C-fiber afferents in monkeys with human judgments of thermal pain. J Neurophysiol. 1978;41:509–528. [PubMed: 418156]
- Launay P, Fleig A, Perraud AL, Scharenberg AM, Penner R, Kinet JP. TRPM4 is a Ca2+-activated nonselective cation channel mediating cell membrane depolarization. Cell. 2002;109:397–407. [PubMed: 12015988]
- Lee H, Iida T, Mizuno A, Suzuki M, Caterina MJ. Altered thermal selection behavior in mice lacking transient receptor potential vanilloid 4. J Neurosci. 2005;25:1304–1310. [PMC free article: PMC6725965] [PubMed: 15689568]
- Lewinter RD, Skinner K, Julius D, Basbaum AI. Immunoreactive TRPV-2 (VRL-1), a capsaicin receptor homolog, in the spinal cord of the rat. J Comp Neurol. 2004;470:400–408. [PubMed: 14961565]
- Liedtke W, Choe Y, Marti-Renom MA, Bell AM, Denis CS, Sali A, Hudspeth AJ, Friedman JM, Heller S. Vanilloid receptor-related osmotically activated channel (VR-OAC), a candidate vertebrate osmoreceptor. Cell. 2000;103:525–535. [PMC free article: PMC2211528] [PubMed: 11081638]
- Liedtke W, Friedman JM. Abnormal osmotic regulation in trpv4−/− mice. Proc Natl Acad Sci USA. 2003;100:13698–13703. [PMC free article: PMC263876] [PubMed: 14581612]
- Liu B, Qin F. Functional control of cold- and menthol-sensitive TRPM8 ion channels by phosphatidylinositol 4,5-bisphosphate. J Neurosci. 2005;25:1674–1681. [PMC free article: PMC6725927] [PubMed: 15716403]
- Ma QP. Vanilloid receptor homologue, VRL1, is expressed by both A- and C-fiber sensory neurons. Neuroreport. 2001;12:3693–3695. [PubMed: 11726776]
- Macpherson LJ, Geierstanger BH, Viswanath V, Bandell M, Eid SR, Hwang S, Patapoutian A. The pungency of garlic: activation of TRPA1 and TRPV1 in response to allicin. Curr Biol. 2005;15:929–934. [PubMed: 15916949]
- McKemy DD, Neuhausser WM, Julius D. Identification of a cold receptor reveals a general role for TRP channels in thermosensation. Nature. 2002;416:52–58. [PubMed: 11882888]
- Mizumura K, Kumazawa T. Modification of nociceptor responses by inflammatory mediators and second messengers implicated in their action—a study in canine testicular polymodal receptors. Prog Brain Res. 1996;113:115–141. [PubMed: 9009731]
- Moqrich A, Hwang SW, Earley TJ, Petrus MJ, Murray AN, Spencer KS, Andahazy M, Story GM, Patapoutian A. Impaired thermosensation in mice lacking TRPV3, a heat and camphor sensor in the skin. Science. 2005;307:1468–1472. [PubMed: 15746429]
- Moriyama T, Higashi T, Togashi K, Iida T, Segi E, Sugimoto Y, Tominaga T, Narumiya S, Tominaga M. Sensitization of TRPV1 by EP1 and IP reveals peripheral nociceptive mechanism of prostaglandins. Molecular Pain. 2005;1:3–12. [PMC free article: PMC1074353] [PubMed: 15813989]
- Moriyama T, Iida T, Kobayashi K, Higashi T, Fukuoka T, Tsumura H, Leon C, Suzuki N, Inoue K, Gachet C, Noguchi K, Tominaga M. Possible involvement of P2Y2 metabotropic receptors in ATP-induced transient receptor potential vanilloid receptor 1-mediated thermal hypersensitivity. J Neurosci. 2003;23:6058–6062. [PMC free article: PMC6740351] [PubMed: 12853424]
- Nagy I, Rang HP. Similarities and differences between the responses of rat sensory neurons to noxious heat and capsaicin. J Neurosci. 1999;19:10647–10655. [PMC free article: PMC6784946] [PubMed: 10594048]
- Nealen ML, Gold MS, Thut PD, Caterina MJ. TRPM8 mRNA is expressed in a subset of cold-responsive trigeminal neurons from rat. J Neurophysiol. 2003;90:515–520. [PubMed: 12634279]
- Nilius B, Prenen J, Droogmans G, Voets T, Vennekens R, Freichel M, Wissenbach U, Flockerzi V. Voltage dependence of the Ca2+-activated cation channel TRPM4. J Biol Chem. 2003;278:30813–30820. [PubMed: 12799367]
- Numazaki M, Tominaga T, Toyooka H, Tominaga M. Direct phosphorylation of capsaicin receptor VR1 by protein kinase C epsilon and identification of two target serine residues. J Biol Chem. 2002;277:13375–13378. [PubMed: 11884385]
- Obata K, Katsura H, Mizushima T, Yamanaka H, Kobayashi K, Dai Y, Fukuoka T, Tokunaga A, Tominaga M, Noguchi K. TRPA1 induced in sensory neurons contributes to cold hyperalgesia after inflammation and nerve injury. J Clin Invest. 2005;115:2393–2401. [PMC free article: PMC1187934] [PubMed: 16110328]
- Patapoutian A, Peier AM, Story GM, Viswanath V. ThermoTRP channels and beyond: mechanisms of temperature sensation. Nat Rev Neurosci. 2003;4:529–539. [PubMed: 12838328]
- Peier AM, Moqrich A, Hergarden AC, Reeve AJ, Andersson DA, Story GM, Earley TJ, Dragoni I, McIntyre P, Bevan S, Patapoutian A. A TRP channel that senses cold stimuli and menthol. Cell. 2002;108:705–715. [PubMed: 11893340]
- Peier AM, Reeve AJ, Andersson DA, Moqrich A, Earley TJ, Hergarden AC, Story GM, Colley S, Hogenesch JB, McIntyre P, Bevan S, Patapoutian A. A heat-sensitive TRP channel expressed in keratinocytes. Science. 2002;296:2046–2049. [PubMed: 12016205]
- Perez CA, Huang L, Rong M, Kozak JA, Preuss AK, Zhang H, Max M, Margolskee RF. A transient receptor potential channel expressed in taste receptor cells. Nat Neurosci. 2002;5:1169–1176. [PubMed: 12368808]
- Prawitt D, Enklaar T, Klemm G, Gartner B, Spangenberg C, Winterpacht A, Higgins M, Pelletier J, Zabel B. Identification and characterization of MTR1, a novel gene with homology to melastatin (MLSN1) and the trp gene family located in the BWS-WT2 critical region on chromosome 11p15.5 and showing allele-specific expression. Hum Mol Genet. 2000;9:203–216. [PubMed: 10607831]
- Prawitt D, Monteilh-Zoller MK, Brixel L, Spangenberg C, Zabel B, Fleig A, Penner R. TRPM5 is a transient Ca2+-activated cation channel responding to rapid changes in [Ca2+]i. Proc Natl Acad Sci USA. 2003;100:15166–15171. [PMC free article: PMC299937] [PubMed: 14634208]
- Premkumar LS, Raisinghani M, Pingle SC, Long C, Pimentel F. Downregulation of transient receptor potential melastatin 8 by protein kinase C–mediated dephosphorylation. J Neurosci. 2005;25:11322–11329. [PMC free article: PMC6725906] [PubMed: 16339027]
- Reichling DB, Levine JD. Heat transduction in rat sensory neurons by calcium-dependent activation of a cation channel. Proc Natl Acad Sci USA. 1997;94:7006–7011. [PMC free article: PMC21275] [PubMed: 9192682]
- Reid G, Babes A, Pluteanu F. A cold- and menthol-activated current in rat dorsal root ganglion neurones: properties and role in cold transduction. J Physiol. 2002;545:595–614. [PMC free article: PMC2290674] [PubMed: 12456837]
- Reid G, Flonta ML. Physiology. Cold current in thermoreceptive neurons. Nature. 2001;413:480. [PubMed: 11586349]
- Rohacs T, Lopes CM, Michailidis I, Logothetis DE. PI(4,5)P2 regulates the activation and desensitization of TRPM8 channels through the TRP domain. Nat Neurosci. 2005;8:626–634. [PubMed: 15852009]
- Shimosato G, Amaya F, Ueda M, Tanaka Y, Decoster I, Tanaka M. Peripheral inflammation induces up-regulation of TRPV2 expression in rat DRG. Pain. 2005;119:225–232. [PubMed: 16298071]
- Simone DA, Kajander KC. Responses of cutaneous A-fiber nociceptors to noxious cold. J Neurophysiol. 1997;77:2049–2060. [PubMed: 9114254]
- Smith GD, Gunthorpe MJ, Kelsell RE, Hayes PD, Reilly P, Facer P, Wright JE, Jerman JC, Walhin JP, Ooi L, Egerton J, Charles KJ, Smart D, Randall AD, Anand P, Davis JB. TRPV3 is a temperature-sensitive vanilloid receptor-like protein. Nature. 2002;418:186–190. [PubMed: 12077606]
- Story GM, Peier AM, Reeve AJ, Eid SR, Mosbacher J, Hricik TR, Earley TJ, Hergarden AC, Andersson DA, Hwang SW, McIntyre P, Jegla T, Bevan S, Patapoutian A. ANKTM1, a TRP-like channel expressed in nociceptive neurons, is activated by cold temperatures. Cell. 2003;112:819–829. [PubMed: 12654248]
- Strotmann R, Harteneck C, Nunnenmacher K, Schultz G, Plant TD. OTRPC4, a nonselective cation channel that confers sensitivity to extracellular osmolarity. Nat Cell Biol. 2000;2:695–702. [PubMed: 11025659]
- Sugiura T, Tominaga M, Katsuya H, Mizumura K. Bradykinin lowers the threshold temperature for heat activation of vanilloid receptor 1. J Neurophysiol. 2002;88:544–548. [PubMed: 12091579]
- Suzuki M, Mizuno A, Kodaira K, Imai M. Impaired pressure sensation in mice lacking TRPV4. J Biol Chem. 2003;278:22664–22668. [PubMed: 12692122]
- Talavera K, Yasumatsu K, Voets T, Droogmans G, Shigemura N, Ninomiya Y, Margolskee RF, Nilius B. Heat activation of TRPM5 underlies thermal sensitivity of sweet taste. Nature. 2005;438:1022–1025. [PubMed: 16355226]
- Tillman DB, Treede RD, Meyer RA, Campbell JN. Response of C fibre nociceptors in the anaesthetized monkey to heat stimuli: estimates of receptor depth and threshold. J Physiol. 1995;485(Pt 3):753–765. [PMC free article: PMC1158041] [PubMed: 7562614]
- Todaka H, Taniguchi J, Satoh J, Mizuno A, Suzuki M. Warm temperature-sensitive transient receptor potential vanilloid 4 (TRPV4) plays an essential role in thermal hyperalgesia. J Biol Chem. 2004;279:35133–35138. [PubMed: 15187078]
- Tominaga M, Caterina MJ. Thermosensation and pain. J Neurobiol. 2004;61:3–12. [PubMed: 15362149]
- Tominaga M, Caterina MJ, Malmberg AB, Rosen TA, Gilbert H, Skinner K, Raumann BE, Basbaum AI, Julius D. The cloned capsaicin receptor integrates multiple pain-producing stimuli. Neuron. 1998;21:531–543. [PubMed: 9768840]
- Tominaga M, Wada M, Masu M. Potentiation of capsaicin receptor activity by metabotropic ATP receptors as a possible mechanism for ATP-evoked pain and hyperalgesia. Proc Natl Acad Sci USA. 2001;98:6951–6956. [PMC free article: PMC34459] [PubMed: 11371611]
- Tracey WD Jr, Wilson RI, Laurent G, Benzer S. painless, a Drosophila gene essential for nociception. Cell. 2003;113:261–273. [PubMed: 12705873]
- Treede RD, Meyer RA, Raja SN, Campbell JN. Evidence for two different heat transduction mechanisms in nociceptive primary afferents innervating monkey skin. J Physiol. 1995;483(Pt 3):747–758. [PMC free article: PMC1157815] [PubMed: 7776255]
- Viswanath V, Story GM, Peier AM, Petrus MJ, Lee VM, Hwang SW, Patapoutian A, Jegla T. Opposite thermosensor in fruitfly and mouse. Nature. 2003;423:822–823. [PubMed: 12815418]
- Vlachova V, Teisinger J, Susankova K, Lyfenko A, Ettrich R, Vyklicky L. Functional role of C-terminal cytoplasmic tail of rat vanilloid receptor 1. J Neurosci. 2003;23:1340–1350. [PMC free article: PMC6742269] [PubMed: 12598622]
- Voets T, Droogmans G, Wissenbach U, Janssens A, Flockerzi V, Nilius B. The principle of temperature-dependent gating in cold- and heat-sensitive TRP channels. Nature. 2004;430:748–754. [PubMed: 15306801]
- Watanabe H, Davis JB, Smart D, Jerman JC, Smith GD, Hayes P, Vriens J, Cairns W, Wissenbach U, Prenen J, Flockerzi V, Droogmans G, Benham CD, Nilius B. Activation of TRPV4 channels (hVRL-2/mTRP12) by phorbol derivatives. J Biol Chem. 2002;277:13569–13577. [PubMed: 11827975]
- Watanabe H, Vriens J, Prenen J, Droogmans G, Voets T, Nilius B. Anandamide and arachidonic acid use epoxyeicosatrienoic acids to activate TRPV4 channels. Nature. 2003;424:434–438. [PubMed: 12879072]
- Watanabe H, Vriens J, Suh SH, Benham CD, Droogmans G, Nilius B. Heat-evoked activation of TRPV4 channels in a HEK293 cell expression system and in native mouse aorta endothelial cells. J Biol Chem. 2002;277:47044–47051. [PubMed: 12354759]
- Wissenbach U, Bodding M, Freichel M, Flockerzi V. Trp12, a novel Trp-related protein from kidney. FEBS Lett. 2000;485:127–134. [PubMed: 11094154]
- Wood JN, Perl ER. Pain. Curr Opin Genet Dev. 1999;9:328–332. [PubMed: 10377285]
- Woolf CJ, Salter MW. Neuronal plasticity: increasing the gain in pain. Science. 2000;288:1765–1769. [PubMed: 10846153]
- Xu H, Ramsey IS, Kotecha SA, Moran MM, Chong JA, Lawson D, Ge P, Lilly J, Silos-Santiago I, Xie Y, DiStefano PS, Curtis R, Clapham DE. TRPV3 is a calcium-permeable temperature-sensitive cation channel. Nature. 2002;418:181–186. [PubMed: 12077604]
- Zars T. Hot and cold in Drosophila larvae. Trends Neurosci. 2003;26:575–577. [PubMed: 14585595]
- Review [Molecular mechanisms of thermosensation].[Nihon Yakurigaku Zasshi. 2004]Review [Molecular mechanisms of thermosensation].Tominaga M. Nihon Yakurigaku Zasshi. 2004 Oct; 124(4):219-27.
- Review Thermosensation and pain.[J Neurobiol. 2004]Review Thermosensation and pain.Tominaga M, Caterina MJ. J Neurobiol. 2004 Oct; 61(1):3-12.
- Review ThermoTRP channels as modular proteins with allosteric gating.[Cell Calcium. 2007]Review ThermoTRP channels as modular proteins with allosteric gating.Latorre R, Brauchi S, Orta G, Zaelzer C, Vargas G. Cell Calcium. 2007 Oct-Nov; 42(4-5):427-38. Epub 2007 May 17.
- Review Osmomechanical-Sensitive TRPV Channels in Mammals.[Neurobiology of TRP Channels. ...]Review Osmomechanical-Sensitive TRPV Channels in Mammals.Moore C, Liedtke WB. Neurobiology of TRP Channels. 2017
- Review TRPV1 Receptors and Signal Transduction.[TRP Ion Channel Function in Se...]Review TRPV1 Receptors and Signal Transduction.Rosenbaum T, Simon SA. TRP Ion Channel Function in Sensory Transduction and Cellular Signaling Cascades. 2007
- The Role of TRP Channels in Thermosensation - TRP Ion Channel Function in Sensor...The Role of TRP Channels in Thermosensation - TRP Ion Channel Function in Sensory Transduction and Cellular Signaling Cascades
Your browsing activity is empty.
Activity recording is turned off.
See more...